Cell-based immunotherapy
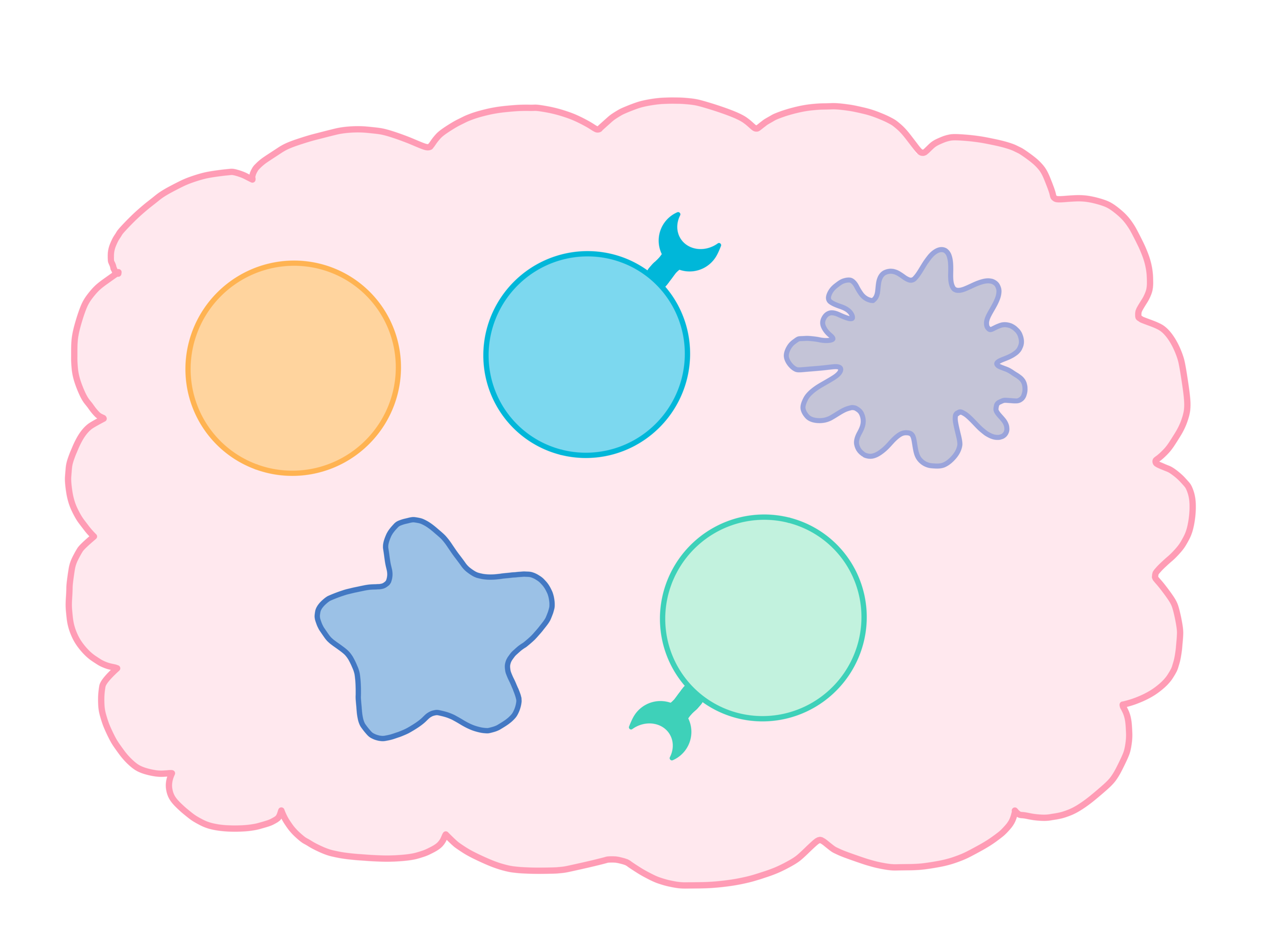
What is cell-based immunotherapy?
Cell-based cancer immunotherapies are treatments that involve delivering living immune cells into a patient to fight their cancer. In some cases, the immune cells are collected from the patient and are a perfect genetic match, while in other cases, they are collected from a family member or other well matched healthy donor. Often, the living cells are enriched for certain cell types and/or are modified in a research laboratory or specialized manufacturing facility in order to maximize their ability to fight the cancer before they are given to the patient.
There are several different types of cell-based immunotherapies. Historically, they have been used to restore the immune systems of patients treated with high-intensity radiation and/or chemotherapy, which, in addition to killing the cancer cells, also destroys healthy blood and bone marrow cells – the source of the body's blood and immune cells. However, as scientists and clinicians have learned more about the important role the immune system plays in fighting cancer, new strategies have been developed to produce and deliver specialized, or even genetically engineered immune cells as treatments for a variety of cancers.
The sections below describe the major types of cell-based immunotherapy currently being used, as well as promising new therapies currently under investigation.
T Cell Therapy
T cells are considered the “warriors of the immune system”, especially when it comes to the fight against cancer. Cancer cells often make abnormal molecules, known as cancer antigens, that T cells are able to recognize. Upon recognition of these antigens, the T cells seek and destroy the cancer cells that carry them. The aim of T cell therapy is to collect some of a patient’s own T cells from their body, grow them in a laboratory to increase their number, and then deliver high numbers of living T cells back into the patient in order to enhance the T cell response against cancer compared to what would occur naturally. In the laboratory, T cells may be selected based on certain qualities, multiplied, enhanced in existing capabilities, and/or endowed with new functions or features using genetic engineering. Each of these strategies can potentially improve their cancer-killing functions. Several types of T cell therapies are approved for use in the clinic for certain blood cancers, with patients often achieving positive outcomes. Please visit our section on T cell Therapy to learn more.
Natural Killer (NK) Cell Therapy
Natural killer (NK) cells are an important type of immune cell that circulates throughout the body. They recognize cells that appear infected, altered, damaged, or stressed out, and are readily armed with the tools to attack and kill such cells. NK cells are considered a part of the innate immune system, which includes many of the first responders to any abnormal cells or activity in the body, and is quick to respond to any potential threats.
NK cells become activated based on a balance of activating (“something's wrong!”) or inhibiting (“everything is ok!”) signals they receive from cells and molecules in their environment. Activating signals predominate when there is an infection or tissue damage that needs cleanup and repair. In the case of cancer, unusual proteins on the surface of the cancerous cell can trigger the activating signals, shifting the signaling balance enough to initiate an NK cell attack. In addition to killing cancerous cells on site, NK cells send out additional signals that recruit and activate other immune cells, such as dendritic cells, which go on to recruit cells of the adaptive immune system, which are stronger and more specialized, but take more time to respond.
While NK cells have been shown to have strong antitumor activity, and high levels of NK cells in tumors have been linked to increased patient survival, NK cells are typically only found at low numbers in human tumors, as tumors often find ways to keep NK cells out. In NK cell-based immunotherapy, NK cells are usually collected from blood, multiplied in a laboratory, and delivered to a patient in high numbers in order to increase NK cell killing of cancer cells. Unlike other types of immune cells, NK cells are less specific to an individual, so they do not need to be genetically matched to a particular patient; they can come from almost any donor, and be given to the patient with minimal risk of unwanted attack of healthy tissue.
For use in therapies, NK cells are collected from a patient’s or donor’s blood via leukapheresis, obtained from umbilical cord blood, or grown from specialized stem cells. The NK cells are then multiplied in the lab, and in some cases, additional factors (e.g., cytokines) can be used to activate them, enhance their functions, or lengthen their lifespan. In addition, NK cells may be altered in the laboratory using drugs or genetic engineering to enhance their activation status or functions.
Prior to treatment with an NK cell product, patients are usually treated with one or more powerful chemotherapeutic drugs, and sometimes low-dose radiation, to eliminate as many of the cancer cells as possible, and to reduce the numbers of the patient’s natural immune cells. With fewer patient immune cells in the blood, the laboratory-made cells don’t have to compete for resources, improving their chances of surviving, multiplying, and attacking cancer cells. This treatment phase is called “lymphodepletion” or “conditioning”, and it usually occurs shortly before administration of the NK cell therapy. Sometimes patients are also pre-treated with a drug that makes cancer cells more sensitive to NK cell killing over the course of approximately two weeks before receiving the NK cell product. Treatment with NK cells involves the delivery of a large number of NK cells through a tube in the vein (infusion). Once inside the patient, NK cells may continue to multiply and survive, thereby acting as a living drug that can kill cancer cells wherever they are found.
Recently, researchers have used genetic engineering to create modified NK cells with artificial receptors called chimeric antigen receptors, or CARs, that recognize target molecules on the cancer cells. The resulting CAR NK cells are able to directly bind to cancer cells that may otherwise have escaped recognition by NK cells, allowing for better targeting and killing of cancers. CAR NK cell therapy is very similar to CAR T cell therapy.
Dendritic Cell Vaccines
Dendritic cells are specialized antigen-presenting immune cells, and are frequently utilized in cancer vaccination. In order to protect the body from a threat, various immune cells, including dendritic cells, move in to launch an early attack once a pathogen (like a virus or bacteria) or cells that are behaving abnormally have been detected. Dendritic cells pick up debris and evidence of the threat (antigens) and carry it to the lymph nodes, where they present the evidence to B cells (which release antibodies), and T cells (the warriors of the immune system). If the T cells or B cells recognize the evidence presented by the dendritic cells, they will begin to launch a strong and highly specific attack against the threat.
In cancer immunotherapy, dendritic cells can be used as vaccines. To do this, researchers collect them from the patient’s blood, grow them in a laboratory, “load” them with select antigens, and activate them to enhance their ability to initiate strong immune responses. These “loaded” and activated dendritic cells can then be injected back into the patient, where they go on to activate strong B and T cell responses to the antigens they now carry. Please visit our section on cancer vaccines to learn more.
Cytokine-Induced Killer (CIK) and Other Cell-Based Immunotherapies
Many different immune cell types and cell modification strategies have been developed for use in cancer immunotherapy, and many have been or are currently being tested. For example, cytokine-induced killer cell (CIK) and lymphokine-activated killer cell (LAK) therapies consist of a mix of immune cells with features of natural killer T (NKT) cells or natural killer (NK) cells that can target and eliminate cancer cells. These therapies are generated by growing blood and immune cell-forming hematopoietic stem cells with molecules (e.g., cytokines) that can induce their development and maturation in a laboratory. Cell therapies that utilize macrophages, invariant natural killer cells (iNKT) cells and ɣẟT cells are also being explored. Further, genetic engineering can be used to add or delete genes from virtually any immune cells to enhance their potential cancer-fighting functions, opening up the door for many more cell-based immunotherapies moving forward.
Hematopoietic Stem Cell Transplantation (HSCT)
Hematopoietic stem cell transplantation (HSCT) is the oldest form of cell-based immunotherapy. Hematopoietic stem cells are cells that primarily reside in bone marrow (the sponge-like substance inside of bones) and give rise to new red blood cells, immune cells, and platelets that go on to circulate throughout the body. A hematopoietic stem cell transplant involves transferring healthy hematopoietic stem cells to a patient in order to rebuild their blood and immune system, usually after it has been weakened or destroyed due to cancer or aggressive cancer treatment.
Stem cell transplantation has become a standard part of treatment for several cancers, especially blood cancers, such as advanced leukemia, lymphoma, or multiple myeloma. These cancers reside primarily in the blood and/or bone marrow and disrupt immunity. They often require treatments that can wipe out the stem, blood, and immune cells of the patient. Stem cell transplantation is also sometimes used in patients with blood cancer who have no apparent cancer burden remaining (they are in remission), but are at very high risk for the cancer to come back (relapse).
Types of stem cell transplant
Preparing a stem cell product
Conditioning
Treatment with a stem cell product
Graft-versus-Leukemia effect
Graft-versus-Host Disease
Transplant rejection
Types of stem cell transplant
In some cases, the stem cells that are given during HSCT are “autologous” stem cells, meaning they come from the patient themself. Autologous stem cells are collected from the patient’s blood or bone marrow prior to treatment with aggressive chemotherapy and/or radiation. However, some patients may not have a sufficient supply of robust, healthy stem cells due to damage caused by their cancer or from prior aggressive cancer therapies. In these cases, patients may instead receive an “allogeneic” stem cell transplantation, in which the stem cells come from a genetically well matched healthy donor.
Preparing a stem cell product
Preparation of a stem cell transplant involves collecting hematopoietic stem cells (HSCs) from the patient or from a matched healthy donor by one of three methods. The collection procedures are all safe, and there are very rarely any complications. They each have slight advantages and disadvantages, and a doctor will choose the strategy that is best for each individual donor. In the first method, cells are collected from the donor’s bone marrow in a single in-hospital procedure under general or local anesthesia. A needle is inserted into the marrow of a bone (typically a hip bone), and some of the marrow is removed for preparation. In a second method, blood cells are collected through “apheresis”. In this case, donors are pre-treated with a drug that causes stem cells to move from the bone marrow into the circulating blood. Then, a needle is inserted into a vein in the arm of the donor (much like being hooked up to an i.v.), and blood from the donor gets filtered through a machine that collects the desired cells from the blood and returns the rest of the blood back to the donor. In some cases, this may need to be done several times on consecutive days to obtain enough hematopoietic stem cells. General anesthesia is not required. A third method is to use donor stem cells prepared from umbilical cord blood, which is collected after a baby is born and stored in cord blood banks.
Conditioning
Once cells for the transplant have been collected and prepared, the patient is treated with one or more powerful chemotherapeutic drugs, and sometimes radiation, to attempt to eliminate the cancer cells still left in the body. It also kills off most or all hematopoietic stem cells and immune cells in the bone marrow and blood of the patient. This treatment phase is called “conditioning” and occurs shortly before the stem cell transplant is administered. The side effects from this part of the treatment can be severe.
Treatment with a stem cell product
Following conditioning, stem cells are transplanted into the patient through a tube into a vein (infusion). Once in the body, it takes some time for the stem cells to become established and begin replenishing the body’s blood and immune cells. Patients remain at high risk for infection until the new immune system is fully functional. Patients typically stay in the hospital for 2-4 weeks following their transplant. During this time, patients often require transfusions of red blood cells (to prevent anemia) and platelets (to prevent uncontrolled bleeding). They may also receive antibiotics to treat or prevent infections. Infections are most likely to occur in the first three months after the transplant.
Graft-versus-leukemia effect
Allogeneic stem cell transplants may not only restore the patients’ blood cells and immune system with cells that have not been weakened by treatment or cancer, but also provide additional benefits in patients. Donor immune cells that were transferred or “grafted” into the patient’s body may recognize and attack any cancer cells remaining in the patient. This “Graft-versus-Leukemia” effect (GvL) has been shown to enhance cure rates and reduce the risk that the cancer will come back.
Several strategies have been developed, and others are being tested, to further enhance the GvL effect. For example, treating a transplanted patient with cytokines, immune checkpoint inhibitors, or cancer vaccines can help the transplanted immune system to thrive within the patient, increasing the lifespan, number, and function of any anti-cancer immune cells. In some cases, patients may receive an additional treatment called a Donor Lymphocyte Infusion (DLI) after stem cell transplantation in order to boost GvL activity. This procedure involves isolating healthy immune cells – in particular, lymphocytes (such as T cells, B cells or NK cells) – from the blood of the donor and delivering these to the patient.
Graft-versus-Host Disease
While patients who receive allogeneic HSCs may benefit from the number and strength of cells from healthy donors, and from the Graft-versus-Leukemia effect, they are also at a higher risk for Graft-versus-Host Disease (GvHD). GvHD occurs when transplanted cells (the “graft”), which are not a perfect match to the patient, begin to identify the patient’s normal cells (the “host”) as abnormal or foreign and begin destroying healthy tissue. GvHD can cause harm to the patient, and may need to be addressed with additional treatments. The degree of GvHD varies between patients and depends on how closely matched the donor and recipient are. Typically, having a donor who is closely genetically matched lowers the risk of GvHD. Several strategies have been developed, and others are being tested, to minimize GvHD while supporting the clinical benefits of stem cell transplantation and the Graft-versus-Leukemia effect.
Transplant rejection
Although patients’ natural immune systems are often weakened or destroyed by cancer or by the chemotherapy/radiation therapy that occurs prior to HSCT, some of the patient’s own immune cells may remain in the body, and may recognize newly transplanted or “grafted” cells as unfamiliar or foreign, leading to transplant rejection. This can render the transplant ineffective – a serious and potentially life-threatening condition for the patient that needs to be addressed.
Further information
UCIR news story
- Patient 3737: An Early Story of Success in Cancer Immunotherapy
- Can a cancer vaccine keep ovarian cancer at bay?
Scientific references
- Identifying and Targeting Human Tumor Antigens for T Cell-Based Immunotherapy of Solid Tumors (review article) Leko V, Rosenberg SA. Cancer Cell (2020)
- Hematopoietic Stem Cell Transplantation - 50 Years of Evolution and Future Perspectives (review article) Henig I, Zuckerman T. Rambam Maimonides Medical Journal (2014)
- The NK cell–cancer cycle: advances and new challenges in NK cell–based immunotherapies (review article) Bald T, et al. Nature Immunology (2020)
- T cell receptor‐based cancer immunotherapy: Emerging efficacy and pathways of resistance (review article) Chandran SS, Klebanoff CA. Immunological Reviews (2019)